Quantum
bit withstands noise
By
Eric Smalley,
Technology Research News
The everyday world we see around us rests
on a foundation of atoms and subatomic particles and the interactions
among them. Separated from the world at large, these particles behave
according to a very different set of rules than the laws of physics we
experience.
Physicists have been able to study quantum mechanics by isolating particles
for fleeting moments, and what they have found has led some to devise
schemes to make extraordinarily powerful computers by harnessing the bizarre
behavior of the particles.
The main challenge to making useful quantum computers
is being able to isolate the delicate particles from environmental energies,
or noise, like radio waves, magnetic fields, and light for more than small
fractions of a second.
"Because it is virtually impossible to isolate a real-world quantum system
from its environment, decoherence is practically ubiquitous," said Lorenza
Viola, a postdoctoral fellow at the Los Alamos National Laboratory. Decoherence
happens when noise from the environment intrudes on quantum particles'
isolation, changing the quantum mechanical properties used to store information
in quantum computing.
A team of researchers from Los Alamos National Laboratory and the Massachusetts
Institute of Technology has come up with a way of fending off decoherence
by using environmental noise rather than trying to block it.
Quantum computers use isolated atoms or subatomic particles to form quantum
bits. Qubits, like
bits in classical computing, have two positions that can represent the
ones and zeros of binary logic, which is the basis of nearly all computers.
For instance, an electron can be used as a qubit because, like a top,
it can spin in one of two directions: spin up or spin down.
One of the strange qualities of quantum particles is that when a particle
is isolated from its environment, which by definition means it cannot
be observed directly, it acts differently from a particle that is not
isolated and can be observed. An isolated particle is in superposition,
which is some unknown mixture of all possible states. For example, an
electron in superposition could be 1 percent spin up and 99 percent spin
down or 50 percent spin up and 50 percent spin down.
The advantage of using a string of qubits in superposition to represent
data is that it can effectively represent many numbers at once. A string
of seven qubits could represent all 128 of the seven-digit combinations
of spin up and spin down. Ten qubits could represent 1,024 combinations,
and 15 qubits, 32,768 combinations at once. Classical bits can represent
the same number of combinations, but must go through the combinations
one at a time to find, for example, a combination that represents a solution
to a problem.
One way of preserving qubits long enough for them to perform these useful
computations is to control environmental noise in a way that leaves sheltered
zones where some qubits can be protected. "If one has a bunch of qubits
and, say, the noise affects all but the first qubit, then the information
carried by qubit one is clearly preserved," said Viola.
One way of doing this is by making one logical qubit out of the interactions,
or waves generated by several particles, rather than protecting the data
in one physical qubit.
Quantum particles behave like both particles and waves. The geometrical
shape of a particle's wave, its wave function, can be symmetrical, just
like the left and right sides of a person. Interacting particles have
a common wave shape, and when noise from the environment affects all of
the particles equally, the shape of their collective wave contains symmetries.
The Los Alamos and MIT team has made a type of sheltered zone called a
noiseless subsystem that makes a qubit out of the symmetries in the collective
wave function of a set of three carbon atoms.
Using the wave function symmetries of quantum particles to store information
requires more than one particle to represent a logical qubit, but that
qubit preserves quantum information in the face of noise better than a
qubit made of a characteristic like spin in a single particle. In fact,
the noise that produces wave symmetries would be enough to destroy any
single-particle qubit.
An earlier scheme demonstrated by the researchers at the National Institute
of Standards and Technology (NIST) also uses sheltered zones and makes
logical qubits from wave symmetries. The decoherence-free subspaces scheme
uses noise to produce symmetries that do not otherwise affect the underlying
set of particles. The scheme is also less complicated than noiseless subsystems,
but it requires more precisely controlled noise, said Viola. They also
require at least four particles per qubit, while the noiseless subsystem
needs only three.
The noise that produces the wave symmetries in the noiseless subsystem
scheme does change the quantum characteristics like spin in the underlying
set of particles, which makes them more difficult to produce than decoherence-free
subspace qubits, said Daniel Lidar, an assistant professor of chemistry
at the University of Toronto.
On the other hand, noise that affects the particles is more common than
noise that does not, which means noiseless subsystem qubits are potentially
easier to sustain in the real world, said Viola. "Assuming the ability
to identify or engineer quantum devices with the correct symmetries, noiseless
subsystems would allow for more options in implementing robust [quantum]
memories simply because noiseless subsystems are more common than decoherence-free
subspaces," she said.
In addition, noiseless subsystems can be combined with a broader range
of quantum error correction codes than decoherence-free subspaces, Viola
said. Error correction codes catch errors that occur when one or more
bits accidentally change from a one to a zero or vice versa.
The research is a significant step towards robust scalable quantum computing,
said Lidar. As expected, noiseless subsystems produce a significant increase
in coherence time for noise of arbitrary strength, he said. "The result
holds for engineered noise, and hence its utility in real life remains
to be seen," he said.
Although fewer particles are needed to encode qubits using noiseless subsystems
than decoherence-free subspaces, the encoding process is somewhat more
complicated, Lidar said. "A very valuable lesson learned from the [research]
is that the encoding/decoding steps can take a significant amount of time
and contribute to coherence degradation," he said.
The issue of number of particles versus ease of encoding presents researchers
with a trade-off to consider when choosing between noiseless subsystems
and decoherence-free subspaces, said Paul Kwiat, a physics professor at
the University of Illinois at Urbana Champaign. "The trade-off of which
is easier to deal with will depend on the particular system used for quantum
computing."
Though the concept of noiseless subsystems might be important in developing
quantum computers, the particular noiseless subsystem the Los Alamos and
MIT researchers produced is not practical because it was created using
Nuclear Magnetic Resonance (NMR) techniques, he said. NMR, which is also
used for medical imaging, uses strong magnetic fields to align atoms.
NMR quantum computing "is now accepted not to be true quantum computing"
because NMR quantum computers cannot be made with more than a few qubits,
and they also cannot produce quantum entanglement, said Kwiat.
Particles in superposition can also be linked, or entangled, so that they
share one or more quantum characteristics like spin; if environmental
noise knocks an electron out of superposition and into the spin up position,
an electron that was entangled with it also leaves superposition in the
same spin up position, regardless of the physical distance between them.
Entanglement would give practical quantum computers the ability to do
many calculations efficiently.
The technique can be used in other quantum computer architectures, including
trapped ions and solid-state quantum chips, according to Viola.
Practical applications of quantum information processing for cryptography
and simulating quantum mechanics could be achieved in the next few years,
said Viola. However, full-blown quantum computers that are able to, for
example, improve on current capabilities for factoring large numbers are
probably more than 20 years off, she said.
Viola's research colleagues were Emanuel Knill and Raymond Laflamme of
Los Alamos National Laboratory and Evan M. Fortunato, Marco A. Pravia
and David G. Cory of the Massachusetts Institute of Technology. They published
the research in the September 14, 2001 issue of the journal Science. The
research was funded by the Department of Energy, the National Security
Agency (NSA), the Army Research Office and the Defense Advanced Research
Projects Agency (DARPA).
Timeline: 2 years, > 20 years
Funding: Government
TRN Categories: Quantum Computing
Story Type: News
Related Elements: Technical paper, "Experimental Realization
of Noiseless Subsystems for Quantum Information Processing," Science,
September 14, 2001
Advertisements:
|
September
26, 2001
Page
One
Statistics sniff out
secrets
Quantum bit withstands
noise
Image search sorts by
content
Study finds Web quality
time
Powerless memory gains
time
News:
Research News Roundup
Research Watch blog
Features:
View from the High Ground Q&A
How It Works
RSS Feeds:
News | Blog
| Books 
Ad links:
Buy an ad link
Advertisements:
|
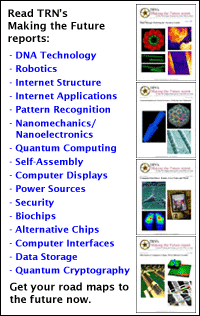
|
|
|