Semiconductors
control quantum spin
By
Eric Smalley,
Technology Research News
An electron is like an infinitesimal top
spinning either clockwise or counterclockwise. The two directions can
represent the ones and zeros of computing, which has tempted researchers
to find new ways to build computers.
The possibilities range from ultra-powerful quantum
computers to ordinary computers that require far less electricity.
The trick is being able to control the direction of the particles' spin.
A research team based at the University of California at Santa Barbara
has built a semiconductor device that uses an electric field to rapidly
reverse the spin of electrons confined to an area 10,000 times smaller
than the head of a pin. The device can change the spin of an electron
in less than a millionth of a second.
The research shows it is possible to use conventional electronics to construct
a 'spin gate' that controls the electron spin, said David D. Awschalom,
a physics professor at the University of California at Santa Barbara.
The technology can be applied "rapidly, locally and with conventional
technologies," he said.
The key advantage of controlling spin in a semiconductor
device is that thousands or millions of the devices could be combined
to make a new kind of computer processor in the same way that millions
of transistors make up today's computer
chips.
Today's computers use the charge of electrons -- the presence or absence
of an electric current in a circuit -- to represent the ones and zeros
of computing. But in order to store digital information, the ones and
zeros have to be translated into the positive and negative fields of tiny
bits of magnetic material in disk drives.
Spin, however, could be used for both processing and storing information.
Spintronic computers would be much faster than today's computers because
they could store information without using magnetic disk drives, which
are much slower than computer chips, and they would require much less
power. Longer term, controlling electron spin could make it easier to
use the weird quantum properties of the particles to build phenomenally
powerful quantum computers.
The researchers achieved their high degree of control over electron spin
using a quantum well -- a relatively simple microscopic device made from
layers of semiconductor material.
Electrons ordinarily either orbit an atom or hop from one atom to another.
This hopping behavior is what allows electrical current to flow through
a wire. Quantum wells catch electrons in flight and hold them in place.
"Quantum wells are nanometer-scale structures used to trap electrons in
specific locations. They are the basis for many of today's electronic
devices, such as the laser in your CD player," Awschalom said.
The researchers shaped their quantum well like a parabola instead of the
usual box shape, said Awschalom. The quantum well is made from a mixture
of the semiconductors gallium arsenide and aluminum gallium arsenide and
is 100 nanometers wide, or about one-tenth the width of an E. coli bacterium.
A nanometer is one millionth of a millimeter.
The researchers created the parabolic shape by gradually varying the concentration
of aluminum across the quantum well, with the lowest concentration at
the center and the highest at the edges. The researchers moved electrons
within a well by turning on an electric field and varying its strength.
The speed and direction of an electron's spin in the quantum well is related
to the concentration of aluminum; by moving electrons to specific positions
in the well the researchers were able to speed up, stop and reverse their
spins. Most importantly, the researchers were able to move the electrons
without changing their wave functions, which could allow the electrons
to serve as a quantum bit, or qubit, said Awschalom.
One of the weird aspects of quantum physics is that when an electron is
isolated from its environment it is in superposition, meaning it is in
some mixture of both spin directions and it has some chance of being at
any given point in the quantum well. The mathematical map of these possibilities
is the particle's wave function. When the electron is observed or otherwise
comes into contact with its environment, it's wave function collapses
and it assumes a definite position and spin direction.
Multiple particles in the same space have a combined wave function, which
is the case for the electrons in the researchers' quantum well.
The challenge for quantum computing is to preserve this fragile state
of superposition while manipulating it to perform computations. In the
researchers' device, moving the electrons changes their spins, which is
the manipulation needed for computing, but preserves the wave function.
The reward for achieving precise control of particle spin is tremendous.
A series of qubits that are in superposition can represent every binary
number that has as many or fewer digits than the number of qubits. For
example, three qubits can represent eight different binary numbers and
25 qubits can represent 33,554,432 binary numbers.
The wave functions of a series of qubits can also be linked, or entangled.
When changes are made to one entangled particle, they all change the same
way regardless of the physical distance between them, as long as they
remain in superposition.
Using this bizarre property, quantum computers could examine every possible
answer to a problem with one series of operations rather than having to
check each individually, which means they could solve problems that would
be impossible for the most powerful classical computer conceivable.
Quantum computing algorithms are sequences of single-qubit and two-qubit
operations. The single-qubit operation -- reversing the spin of an electron
-- is essentially what the researchers' spin gate does, said Michael E.
Flatté, an associate professor of physics at the University of Iowa. Though
the researchers have not demonstrated single-qubit operations yet, "their
work indicates a plausible path to them," he said.
The researchers' next major objective is to entangle an array of qubits,
said Awschalom. Entangling at least two qubits would allow for two-qubit
operations; large numbers of entangled qubits would be necessary to make
a practical quantum computer.
The spin gate could be used in practical applications in 10 to 20 years,
said Awschalom.
Awschalom's research colleagues were Gian Salis, Yuichiro Kato, Dan C.
Driscoll and Art C. Gossard of the University of California at Santa Barbara,
and Klaus Ensslin of the Swiss Federal Institute of Technology. They published
the research in the December 6, 2001 issue of the journal Nature. The
research was funded by the Defense Advanced Research Projects Agency (DARPA),
the Office of Naval Research and the National Science Foundation (NSF).
Timeline: 10-20 years
Funding: Government
TRN Categories: Materials Science and Engineering; Quantum
Computing
Story Type: News
Related Elements: Technical paper, "Electrical control of
spin coherence in semiconductor nanostructures," Nature, December 6, 2001
Advertisements:
|
December
12, 2001
Page
One
E-paper coming into view
Semiconductors
control quantum spin
Cold electrons crystallize
Neat not always organized
Single molecule
drives transistor
News:
Research News Roundup
Research Watch blog
Features:
View from the High Ground Q&A
How It Works
RSS Feeds:
News | Blog
| Books 
Ad links:
Buy an ad link
Advertisements:
|
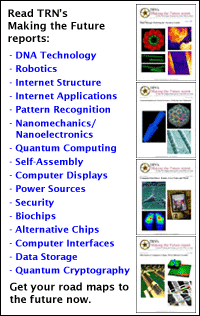
|
|
|