Quantum
scheme lightens load
By
Eric Smalley,
Technology Research News
Two years ago, scientists proved it possible
to build a quantum computer from simple optical equipment commonly found
in university classrooms and laboratories. Now researchers at Johns Hopkins
University have refined the approach, reducing the amount of equipment
linear optical quantum computers would need by about two orders of magnitude.
Quantum computers use the weird nature of particles like atoms, electrons
and photons to perform many computations in parallel. If a big enough
quantum computer could be built, it would far outstrip classical computers
for solving certain problems like cracking secret codes. So far, however,
only the most rudimentary quantum prototypes have been constructed.
The Johns Hopkins plan shows that equipment like mirrors, half mirrors
and phase shifters could be used to make practical, photon-based quantum
computers, said James Franson, principal staff at the Johns Hopkins University
Applied Physics Laboratory and a research professor the university's electrical
and computer engineering department. "Our approach may make it more feasible
to develop a full-scale quantum computer," he said.
Controlling single photons using linear optics equipment is simpler than
manipulating individual or small numbers of atoms or electrons, which
are the basic units of most other quantum computing schemes.
Capturing and manipulating atoms and electrons involves precisely tuned
lasers or magnetic fields, or carefully constructed microscopic devices.
It's also much harder to transport isolated atoms and electrons than it
is to move photons. "An optical approach to quantum computing would have
a number of potential advantages, including the ability to connect different
devices using optical fibers in analogy with the wires of a conventional
computer," said Franson.
Linear optical quantum computers, like ordinary electronic computers,
would use circuits that link simple logic devices in intricate patterns
that make the output from one device the input to the next. The 1s and
0s of linear optical quantum computing would be represented by properties
of photons like horizontal versus vertical polarization rather than the
presence or absence of a current of electrons.
The potential power of any type of quantum computer comes from its ability
to examine all possible solutions to a problem at once rather than having
to check one at a time.
This is possible because when a particle like a photon is isolated from
its environment it is in the weird quantum state of superposition, meaning
it can be horizontally and vertically polarized at once, and so can represent
a mix of 1 and 0. This allows a string of photons in superposition to
represent every combination of 1s and 0s at the same time so that a quantum
computer could process all the numbers that represent possible solutions
to a problem using one set of operations on the single string of photons.
Linear optical devices perform quantum logic operations by altering photons
according to probabilities. Half mirrors, or beam splitters, for example,
can direct photons along one of two paths, with an even chance for each
path.
The challenge of linear optical quantum computing is to pass the correct
result of a quantum logic operation from one device to the next without
directly observing the states of the photons that represent the results,
because this would change the states and therefore destroy the information
the photons contain.
The trick is to put additional photons through the logic operation at
the same time. These additional, ancilla photons trigger the optical circuitry
that passes along the output of the logic operation when the result of
the operation is correct. The ancilla photons are absorbed in photon detectors
in the circuitry, but the output photons are preserved and passed on.
The key advance in the Johns Hopkins researchers' approach is that it
uses fewer ancilla photons by entangling input and ancilla photons in
a way that minimizes the probability of errors, said Franson. When two
or more particles in superposition come into contact with each other,
they can become entangled, meaning one or more of their properties change
in lockstep even if the particles are separated.
Fewer ancilla photons means fewer pieces of equipment are needed. "Using
the current error correction techniques, our high-fidelity approach should
reduce the [equipment] required by roughly two orders of magnitude," said
Franson. The amount of equipment required to generate the entangled ancilla
state and the probability of an error "both increase rapidly with increasing
numbers of ancilla photons," he said.
The original linear optical quantum computing scheme had an average error
rate of 2/n, while the researchers' refined scheme has an average error
rate of 4/n2, according to Franson. N represents the number of ancilla
photons. This translates to error rates of 20 percent versus 4 percent
for 10 ancilla photons, and 2 percent versus 0.04 percent for 100 ancilla
photons.
This gives the Johns Hopkins scheme a practical error rate with far fewer
ancilla photons, said Franson. Quantum error correction will require error
rates on the order of 0.1 to 0.01 percent, he said. "That range of errors
could be achieved with 100 ancilla in our case, but that would require
5,000 ancilla in the original... method."
Because the scheme requires fewer mirrors and beam splitters to manipulate
the smaller number of ancilla photons, it makes it more likely that a
practical linear optical quantum computer could be built, said Jonathan
Dowling, supervisor of the quantum computing technologies group at NASA's
Jet Propulsion Laboratory. The researchers' method "seems to be a substantial
improvement over the original scheme," he said.
Devices enabled by this new approach will be used in quantum communications
systems before they are used in full-blown quantum computers, said Dowling.
With experience gained from making quantum communications devices, the
researchers' approach will eventually lead to "a practical, compact, all-optical
quantum computer," he said.
Dowling's group has developed a plan for a quantum repeater, a device
necessary to boost quantum communications over long distances, that is
based in part on the researchers' linear optical quantum logic, said Dowling.
The researchers have shown that the overhead needed to achieve a given
fidelity for linear optical quantum logic gates can be significantly improved,
said Emanuel Knill, a mathematician at Los Alamos National Laboratory
and one of the scientists who developed the concept of linear optical
quantum computing.
The Johns Hopkins researchers' approach does not address logical qubits,
however, said Knill. Logical qubits are encoded from two or more physical
qubits, and this makes them more resistant to errors. "My preference is
to use logical qubits," said Knill. "If one wishes to use physical, not
logical, qubits, then the authors' approach would help significantly,"
he said.
Quantum repeaters could be developed in five years, said Franson. "Full-scale
quantum computers would be much more difficult and would probably require
15 to 20 years in the most optimistic scenario," he said.
The researchers are working on making photon-based logic gates and memory
devices, and single-photon sources, said Franson. "These are the basic
building blocks of a linear optics approach to quantum computing," he
said.
Franson's research colleagues were Michelle Donegan, Michael Fitch, Bryan
Jacobs, and Todd Pittman. They published the research in the September
23, 2002 issue of the journal Physical Review Letters. The research was
funded by the Office of Naval Research (ONR), the Army Research Office
(ARO), the National Security Agency (NSA) and the Department of Defense
(DOD) Independent Research and Development Program (IR&D).
Timeline: 5 years, 15-20 years
Funding: Government
TRN Categories: Physics Quantum Computing and Communications
Story Type: News
Related Elements: Technical paper, "High-Fidelity Quantum
Logic Operations Using Linear Optical Elements," Physical Review Letters,
September 23, 2002
Advertisements:
|
October
16/23, 2002
Page
One
Chemists brew tiny wires
Voiceprints make crypto
keys
Stamp corrals tiny bits
Net devices arranged
fractally
Quantum scheme lightens
load
News:
Research News Roundup
Research Watch blog
Features:
View from the High Ground Q&A
How It Works
RSS Feeds:
News | Blog
| Books 
Ad links:
Buy an ad link
Advertisements:
|
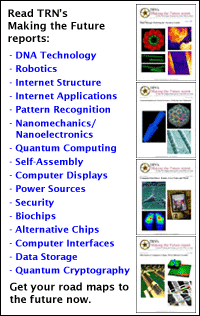
|
|
|