Material
soaks up the sun
By
Kimberly Patch,
Technology Research News
Capturing solar energy efficiently means
finding materials that absorb as many wavelengths of light as possible
that the sun sends our way.
Researchers from Lawrence Berkeley National Laboratory, the University
of California at Berkeley and Cornell University have discovered that
measurements of the semiconductor indium nitride taken two decades ago
were wrong. The measurements led researchers to erroneously classify the
material as a mediocre photovoltaic.
Instead, the material's band gap falls squarely in the solar spectrum,
making it potentially more efficient than currently used photovoltaics.
A material's band gap determines which wavelengths of photons can excite
electrons in the material to create a flow of electricity.
The reclassified material promises to boost the efficiency of solar cells,
allowing for smaller cells or cells of the same size that collect more
electricity.
The best photovoltaic material currently available, a combination of gallium
arsenide and gallium indium phosphide, has a theoretical efficiency of
32 percent; indium nitride has the potential to increase that to 50 percent,
said Wladek Walukiewicz, a senior staff scientist at Lawrence Berkeley
National Laboratory.
The researchers' initial testing also shows that the material withstands
high energy particle irradiation without breaking down. This bodes well
for satellite and other solar collectors that work in space.
When they made their surprising discovery, the researchers were investigating
the mysterious lack of emissions from the material at the conventionally
understood band gap of two electron volts. Instead of finding unusual
reasons for the lack, they discovered that the material was simply misclassified,
and instead has a band gap of 0.7 electron volts. "Once we looked at a
lower energy range we could easily see all the features characteristic
of [a] direct band-gap semiconductor," said Walukiewicz.
The misclassification took place at a time when samples were prepared
by sputtering the material onto a surface. "Such samples contain large
amounts of oxygen, as much as 30 percent. So the samples were indium-oxygen-nitride
alloys rather than indium nitride," said Walukiewicz.
The more modern samples, in contrast, were grown by molecular beam epitaxy,
a process that takes place in a vacuum. "Our material... contains undetectable
levels of oxygen," he said.
A solar cell works by separating the positive and negative charges --
holes and electrons -- produced in a semiconductor unit in the when photons
of sunlight hit it. The atomic structure of the semiconductors used in
solar cells determines how many holes and electrons they can generate.
The efficiency of a material has to do with microscopic measurements:
the band gap, or spacing between the levels electrons occupy in a material,
and the wavelengths of solar radiation.
Materials whose electron level spacing matches up with the wavelengths
of the photons hitting it can absorb the photons. When a material absorbs
a photon, some of the photon's energy causes an electron, which holds
a negative charge, to change to a higher-energy position, leaving behind
a positively-charged hole.
To separate these charges, the substance must be doped, or mixed with
another substance to create separate paths for positive and negative charges.
The separate types of semiconductor are n-type, which guides electrons,
and p-type, which guides holes. "When an electron-hole pair is produced
by a photon... the electron is pulled to the n-type side, and the hole
is pulled to the p-type side," said Walukiewicz.
Because the charges naturally attract each other, the charge separation
creates a change in electric potential, much like rolling a rock up a
hill stores energy that can be released by simply starting it on the path
downhill. In a solar circuit the potential stored by the separated charges
can be released in a current of electricity.
The efficiency of a solar cell depends on how much of the total solar
photon flux, or flow, can be converted into charge carriers, said Walukiewicz.
"For a solar cell made of one semiconductor with a specific energy-gap,
only the photons close to the absorption [range] contribute to the electric
current," he said.
To increase the efficiency, however, solar cells can be made by stacking
several cells of semiconductors with different band gaps to catch the
different light waves. "Although each of the cells will convert the solar
energy only from a limited range of photon energies, all of them together
can make use of more photons," said Walukiewicz.
Indium nitride is important because its band gap sits squarely in the
middle of the light spectrum; this makes it possible to produce gallium-indium-nitride
semiconductors that have any gap within the solar spectrum range, and
makes it possible to put more semiconductors in tandem, said Walukiewicz.
"This is a solar-cell-designer paradise [because] one can maximize...
performance by optimizing the number of cells and their band gaps," he
said.
There's a lot of work to be done before practical solar cells can be made
from indium nitride, however. The researchers have not yet made the p-type
form of the material. "One of the biggest challenges is to make p-type
doped indium nitride," said Walukiewicz. The indications are good, however.
It is theoretically easier to make p-type doped indium nitride than to
do the same with gallium nitride, which has already been done, he said.
Gallium nitride is also a direct band-gap material.
The researchers' next step is to make p-type indium nitride. They are
also working to make p-type gallium indium nitride, he said. And they
are more thoroughly testing the properties of the two materials under
high-energy particle irradiation, he said.
The researchers have only tested a few samples, said Cheng Hsiao Wu, a
professor of electrical and computer engineering at the University of
Missouri at Rolla. The reasons for the measurements are not yet clear;
there could be a mechanism involved other than a different band gap, he
said.
Given the benefit of the doubt, however, a 0.7 reading could be useful,
but finding such a material is a long way from making a solar cell, said
Wu. "For actual solar applications, either the current or the voltage
of each solar cell using a particular [part of the] solar spectrum has
to be matched," and this is a particularly tricky proposition for a full-spectrum
solar cell, he said. If the material works out, however, it "may add another
variety of the solar cell we already have for the full visible spectrum
range," he said.
It will take three to four years to develop indium-nitride-based solar
cell technology, said Walukiewicz.
Walukiewicz's research colleagues were Junqiao Wu and Eugene E. Haller
from the University of California at Berkeley and Lawrence Berkeley National
Laboratory, W. Shan, Kin Man Yu and Joel W. Ager III from Lawrence Berkeley
National Laboratory, and Hai Lu and William J. Schaff from Cornell University.
They published the research in the November 15, 2002 issue of Physical
Review B. The research was funded by the Department of Energy.
Timeline: 3-4 years
Funding: Government
TRN Categories: Semiconductors; Materials Science and Engineering
Story Type: News
Related Elements: Technical paper, "Effects of the Narrow
Band Gap on the Properties of InN," Physical Review B, November 15, 2002.
Advertisements:
|
December
11-25, 2002
Page
One
DNA prefers diamond
Material soaks up the sun
Design links quantum bits
Microscopic mix
strengthens magnet
Laser pulses could
speed memory
News:
Research News Roundup
Research Watch blog
Features:
View from the High Ground Q&A
How It Works
RSS Feeds:
News | Blog
| Books 
Ad links:
Buy an ad link
Advertisements:
|
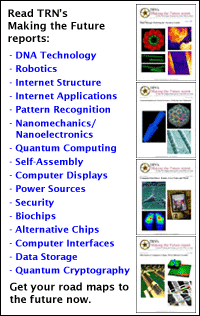
|
|
|