DNA scheme builds computers
By
Kimberly Patch,
Technology Research News
A
decade or so down the road the steady improvements in computer processing
speed that we've grown used to are likely to bump up against the laws of
physics. The lithography used to make the transistors that form computer
circuits uses light and chemicals to etch features into silicon; at some
point the process will not be able to form smaller transistors. Most speed
improvements in computer processors, however, are due to smaller transistors.
One new way to make smaller transistors involves the stuff of life
-- DNA.
Researchers from Duke University, Rambus Inc. and the University
of North Carolina at Chapel Hill have devised a pair of computer architectures
that would be built from self-assembling DNA.
Computers assembled by DNA have the potential to be extremely small,
fast and inexpensive, and would consume very little power.
A single strand of DNA is made up of four bases -- adenine, cytosine,
guanine and thymine -- attached to a sugar-phosphate backbone. Adenine and
thymine bases, and cytosine and guanine bases have the ability to pair up.
Two single strands of DNA whose base orders allow them to completely pair
up form the familiar double helix of biological DNA.
Researchers have tapped the self-assembly ability of DNA by producing
strands of artificial DNA that have segments of base pairs that connect
together in certain patterns. Previous research has shown that it is possible
to coax DNA to self-assemble into three-dimensional structures. DNA can
also be engineered to attach to other materials in order to include those
materials in the self-assembly process.
The researchers' architectures call for single-stranded artificial
DNA molecules that have silicon nanorods attached to their ends to assemble
into circuit patterns. The DNA junctions between rods are then plated with
metal to form the circuitry.
Because the self-assembly process is error prone, the system design
accommodates expected errors by building redundancy into circuitry, said
Dwyer.
The key is to design a system with a large number of simple components
so that any one circuit failure will have a minimal effect on the whole
system. The architectures involve as many as one trillion small processors
working in parallel.
The researchers' approach is distinct from DNA computing research,
which taps the ability of DNA to self-assemble to form strand arrangements
that represent computations. In contrast, the researchers' DNA architectures
harness DNA self-assembly to form nanoelectronic circuits.
The challenge in designing the architectures was designing circuitry
that could self-assemble and devising ways to evaluate the entire system,
said Dwyer. "This work introduces the tools to do this along with two self-assembled
computer architectures," he said.
What the researchers came up with as viable architectures look like
relics from the early infancy of computing, said Dwyer. "Architectures like
these haven't been used for ages," he said.
The architectures follow decoupled array-multiplexer (DAMP) and
oracle designs, respectively. These are simple parallel processing architectures
that could be used to process large optimization problems like the classic
problem of routing a traveling salesman through multiple cities. This problem
requires a lot of computer power because the possibilities rise exponentially
with each added city; there are billions of possible routes through 15 cities,
for example.
The researchers' decoupled array multi-processor architecture involves
processors that communicate only through a central control unit.
The oracle architecture involves a computer assembled for a particular
problem using DNA to match question-answer pairs. The oracle-based computer
responds to a query with an answer if the query contains the answer's question.
The ultimate goal is a computing infrastructure that far exceeds
today's capabilities in cost, speed, size and power consumption, said Dwyer.
"Self-assembly can do this," he said.
It will be 5 to 10 years before a proof-of-concept self-assembled
DNA computer can be built, and more than 10 years before such a computer
could be ready for practical use, said Dwyer.
Eventually self-assembly could replace photolithography as the best
way to fabricate computing devices, said Dwyer. It may prove more practical,
however, for the two methods to merge into a hybrid fabrication method,
he said.
Dwyer's research colleagues were John Poulton of Rambus Inc., and
Russell Taylor and Leandra Vicci of the University of North Carolina at
Chapel Hill. The work appeared in the October 28, 2004 issue of Nanotechnology.
The research was funded by the National Science Foundation (NSF).
Timeline: > 10 years
Funding: Government
TRN Categories: Architecture; DNA Technologies; Nanotechnology
Story Type: News
Related Elements: Technical paper, "DNA Self-Assembled Parallel
Computer Architectures," October 28, 2004, Nanotechnology
Advertisements:
|
January 12/19, 2005
Page
One
Video organizes paper
Conversations control
computers
DNA scheme builds computers
The History Files:
A Short History of the Computer
Letter to readers
Briefs:
Copy-and-paste goes
natural
RNA tiles form nanopatterns
Input device
tracks muscle tremors
Nano gas turbine designed
Ultrasound
makes blood stand out
Silicon surfaces
speed circuits
Branchy
molecules make precise pores
News:
Research News Roundup
Research Watch blog
Features:
View from the High Ground Q&A
How It Works
RSS Feeds:
News | Blog
| Books 
Ad links:
Buy an ad link
Advertisements:
|
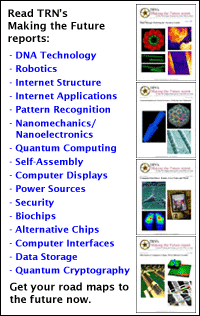
|
|
|