Prototype
transistors promise speedy chips
By
Kimberly Patch,
Technology Research News
Computer chips run faster as the transistors
that make up their logic gates get smaller or in some other way more efficient.
Most chip speed improvements in the last 20 years have been made simply
by shrinking the transistor in order to shorten the path an electrical
signal must travel to turn on a logic gate.
For the past decade or so, however, researchers have been wondering aloud
whether this type of improvement can continue. The two main challenges
are finding ways to etch smaller paths for electrons into the silicon
chips,
and keeping electrons contained within the paths to prevent the volt leakage
that makes chips inefficient.
Researchers at Intel Corp. have gone a long way toward continuing the
improvement until at least the year 2007 with two generations of prototype
transistors that use the common single gate technology found in existing
computers. The prototypes are 30 and 20 nanometers long, and the smaller
one is less than a third the size and more than 10 times faster than those
found on today's chips, according to Gerald Marcyk, director of Intel's
Components Research Lab.
To make the 20-nanometer transistor, the Intel researchers "played some
processing tricks in order to make the lines that small," Marcyk said.
Intel will detail those tricks next year, probably at the Device Research
Conference in Seattle in February, said Marcyk.
Although researchers from the University of California at Berkeley late
last year made a smaller, 15-nanometer prototype that in theory could
be made as small as eight nanometers, it used a more complicated double
gate design.
Conventional transistors have three electrodes. Electrical current enters
through a source electrode on one end of the transistor, exits through
a drain electrode on the other end, and the rate of flow is controlled
by a single gate electrode on top of the device. The double gate design,
with electrodes both on the top and bottom of a transistor, potentially
controls volt leakage better, but makes the devices more difficult to
manufacture.
To keep volt leakage down using the single gate design, the Intel researchers
used less voltage and shrank the layer of silicon oxide used by the control
gate from today's 4-nanometer thickness to 0.8 nanometers. The result
is a three-atom-thick layer of two oxygen and one silicon atom.
The 30 nanometer prototype uses 0.85 volts and will eventually be used
to build 500-million-transistor computer chips that run as fast as 20
gigahertz, or billion cycles per second; the 20 nanometer prototype uses
0.75 volts and will allow for chips that contain up to one billion transistors
and run as fast as 25 gigahertz, Marcyk said.
For comparison, today's Pentium 4 processors use about 42 million 70-nanometer
transistors, 1.3 volts of power to push the electrons through those transistors,
and run as fast as 1.7 gigahertz. The Pentium processors released in 1995
used 5.5 million 2.5 volt, 350-nanometer transistors and had a top speed
of 133 megahertz, or million cycles per second; the 386 processor, first
released in 1985, used 275,000 5-volt, 1,500- and 1,000-nanometer transistors
and had a top speed of 33 megahertz.
Intel's 30- and 20-nanometer transistors are successive generations slated
for use in products in 2005 and 2007, according to Marcyk. Intel's next
generation chip, slated for release in 2003, will use 50-nanometer transistors,
according to Intel.
The researchers are currently working on making these newest generations
of prototypes manufacturable, said Marcyk. "The challenge, of course,
is how you take something from a research project into high-volume manufacturing,"
he said. To make the prototypes, the researchers tweaked the current deep
ultraviolet photolithography
process, which uses 248-nanometer lightwaves. "That's good for making
a handful of transistors to demonstrate a research concept. This is not
a manufacturing process that you take into high-volume and make 100 million
microprocessors," Marcyk said.
To manufacture the 20-nanometer transistors in bulk, the researchers are
looking to another research project, a photolithography process that uses
13-nanometer extreme ultraviolet light that is scheduled to be ready for
practical use in 2006 or 2007, said Marcyk.
In the photolithography process, lightwaves are used to pattern the lines
of a transistor, and the resolution of a line is proportional to the wavelength
of light and the aperture of the lens the light shines through. Current
manufacturing processes use 248-nanometer light. Lab projects have shown
it may be possible to use 157-nanometer light in similar processes, but
that's still not small enough to manufacture 20-nanometer transistors
in bulk, Marcyk said.
One difficulty in using 13-nanometer extreme ultraviolet light is the
light will not go through a lens, and so must be guided using mirrors
instead. Materials like aluminum, however, cannot be part of reflective
surface because they absorb that wavelength of light. "You end up with
a complex multilayer mirror on the surface of the lenses, so there are
some enormous challenges there," said Marcyk.
The first demonstration of a working, 20-nanometer silicon transistor
is an impressive accomplishment that gives the semiconductor industry
a glimmer of hope that alternative transistor structures may not be needed
for several more technology generations, said Tsu-Jae King, an associate
professor of engineering and computer sciences at the University of California
at Berkeley, and director of its microfabrication laboratory. In addition,
extreme ultraviolet lithography shows promise for manufacturing integrated
circuits with 20-nanometer feature sizes, she said. "Cost is the only
real issue."
However, more data, including an assessment of leakage current and information
about how the researchers were able to fashion ultra-shallow source and
drain junctions is needed before a fair assessment can be made about the
transistor's practicality, King said. "The primary challenge in scaling
bulk silicon transistor technology to sub 30-nanometer gate length is
suppression of leakage current," she said.
One solution many in the industry are working on is replacing the silicon
oxide with a thicker layer of a high-K material, which allows electricity
to pass through it more quickly, she said. These materials come with a
challenge, however. "Degradation of [electron] mobility, which results
in degraded drive current, is an issue for many of the high-K dielectrics
being investigated today,” she said.
The Intel researchers are working to find high-K materials in order to
push the size limit further, Marcyk said. "The purpose here is... to extend
the single gate concept as far as possible until it breaks, and then we'll
start using more complex alternatives," said Marcyk. "We have the data
that shows that we can get to 2007 with existing material. And when we
look at the materials and the structures we can clearly go beyond the
end of the decade," he said.
The 20-nanometer prototype is impressive, said Phillip First, an associate
professor of physics at Georgia Tech. One potentially tricky part of making
such small transistors in bulk, however, is keeping down the defect rate,
he said. "When you get down to things that size -- three atoms thick and
20 nanometers -- one of the critical things [is] the success rate because...
a single defect could kill the device, whereas at the larger size scale
you may be able to tolerate a defect within the device and still have
it operate."
At some point there is a limit to how small electron paths can be, First
added. This is because of the split nature of electrons, which act more
like waves than particles as the spaces they're confined to get smaller.
"Ultimately things will have to be designed differently in order to account
for quantum effects -- the wave nature of the electron," he said.
The Intel researchers were Robert Chau, Mark Doczy, Brian Doyle, Pat Stokely,
Dan Lionberger, and Nancy Paulson. Chau presented the research at the
Silicon Nanoelectronics Workshop in Kyoto on June 12, 2001. The research
was funded by Intel.
Timeline: 4-6 years
Funding: Corporate
TRN Categories: Integrated Circuits; Semiconductors
Story Type: News
Related Elements: Technical paper, "30 nm and 20 nm Physical
Gate Length CMOS Transistors," Presented at the Silicon Nano Electronics
Workshop in Kyoto, June 12, 2001.
Advertisements:
|
June
27, 2001
Page
One
Chemists concoct tiny
lasers
Slimmer chips handle
fast nets
Prototype
transistors promise speedy chips
Molecules make short-term
memory
Micromachine parts
relax into place
News:
Research News Roundup
Research Watch blog
Features:
View from the High Ground Q&A
How It Works
RSS Feeds:
News | Blog
| Books 
Ad links:
Buy an ad link
Advertisements:
|
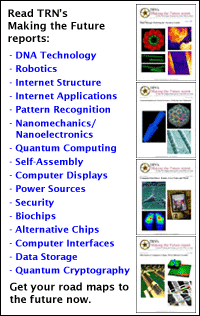
|
|
|