Disks
set to go ballistic
By
Eric Smalley,
Technology Research News
One of the challenges to cramming more
information onto computer hard drives is making a sensor sensitive enough
to measure the presence or absence of a magnetic field in a microscopic
bit of material.
Reading a bit means sensing if its magnetic field affects the flow of
electrons through an electric circuit. If the magnetic field is strong
enough to change a sensor's electron flow, the bit represents a 1, if
not, it is a 0. The smaller the bit, the smaller its magnetic field, and
the harder it is to sense the difference between a 1 and a 0.
Researchers from the State University of New York at Buffalo have used
an effect dubbed ballistic magnetoresistance to make a tiny prototype
sensor that is 15 times as sensitive as the giant magnetoresistance sensors
used in today's disk drives. The T-shaped sensor consists of a pair of
wires connected by only a few hundred atoms.
Because the sensor is very small as well as sensitive, it could be used
to read tiny, closely packed bits. "Due to the nanoscale of the sensor,
bit size can be reduced to... terabit-per-square-inch densities," said
Harsh Deep Chopra, an associate professor of materials science and engineering
at SUNY Buffalo. A terabit is 125 gigabytes, and a DVD holds 4 gigabytes.
A CD-sized magnetic disk with such small bits could hold 1,800 gigabytes,
or about 450 DVDs worth of information.
The key to making sensors that can read smaller bits is increasing the
magnetoresistance of the sensor, or read head, used to distinguish the
magnetic states of the bits. The higher a material's magnetoresistance,
the greater the difference in the number of electrons flowing through
it when it is surrounded by a magnetic field versus when it is not. If
the difference is significant, it can be used to distinguish weak magnetic
fields like those of very small bits that represent 1's from bits that
have no magnetic field and represent 0's.
Ballistic magnetoresistance produces a greater difference between 1 and
0 signals and other types of magnetoresistance, but the challenges to
using it in disk drives is that it works best with either very strong
magnetic fields or extremely low temperatures. Strong magnetic fields
can't be used with small bits, and a low-temperature requirement makes
for impractical devices.
The Buffalo researchers got around these potential restrictions when they
discovered that the right size and shape of the contact point in the tiny
wires of a sensor increases the ballistic magnetoresistance effect to
the point where it can be used with weak magnetic fields at room temperature.
The researchers' ballistic sensor consists of two nickel wires 125 microns
in diameter connected to form a T. A micron is a thousandth of a millimeter,
and a human hair is about 75 microns thick. The researchers sharpened
the end of one wire at the T junction to a 40-nanometer point to make
an extremely small contact between the two wires. A nanometer is one thousandth
of a micron, or about the length of 10 hydrogen atoms in a row.
When the sensor is in a magnetic field, the magnetic orientations of its
electrodes align, making current flow more easily.
In addition, electrons flow even more easily through the sensor because
of its small size. As electrons flow through materials like metal, they
ordinarily scatter, bouncing in different directions. An electrical current
arises when the average of all the movement is a flow in one direction.
In wires only a few nanometers wide, however, the scattering stops and
electrons flow straight through, or ballistically. The sensor allows electrons
to flow this way because the contact surface at the T junction between
the electrodes is only a few hundred atoms
In the absence of a magnetic field, the magnetic orientations of the electrodes
in the researchers' sensor are opposite, which increases the device's
resistance to electrical currents.
The sensor's small size also gives it an an advantage where resistance
is concerned. Electrons have spin, which is similar to a magnet's North
and South poles. If an electron's spin is aligned with the magnetic orientation
of an electrode, it can pass through. If not, it is blocked. If the contact
between the sensor's electrodes were larger, a few electrons would reorient
their spins and pass through, reducing the resistance. But because the
contact is so small, the electrons don't have time to reorient, which
gives the device a higher resistance.
The combination of ballistic electron flow in the presence of a magnetic
field and greater resistance in the absence of a magnetic field means
a greater difference in electron flow than is possible with today's giant
magnetoresistance devices. This makes for a more sensitive sensor.
In low magnetic fields at room temperature, the electrical resistance
of the researchers' sensor is 33 times greater than when no magnetic field
is present, allowing the sensor to read bits so small that one trillion
of them could fit in a square inch, according to Chopra. Previous ballistic
magnetoresistance experiments have yielded increases in resistance of
as much as seven times. The giant magnetoresistance used in today's disk
drives doubles electrical resistance.
Making practical storage devices that use ballistic magnetoresistance
should be fairly straightforward because it is similar to giant magnetoresistance,
said Chopra. Giant magnetoresistance devices use two thin layers of magnetic
material separated by an even thinner non-magnetic layer. Because the
magnetic layers have opposite magnetic orientations, passing a current
of electrons through both layers is difficult: one layer blocks electrons
that have one spin orientation while the other layer blocks electrons
of the other orientation. Adding a magnetic field causes the magnetic
orientations of the two layers to align and so block electrons of only
one spin orientation.
"The good news is that the effect is like giant magnetoresistance except
it is... huge and it comes packed in a sensor size that is atomic scale,"
said Chopra. More work needs to be done to closely control the stronger
ballistic effect and assess its long-term stability, he added.
The device's strong effect at room temperature and in small magnetic fields
makes it "potentially very interesting" for data storage technology, said
Caroline Ross, a professor of materials science and engineering at the
Massachusetts Institute of Technology. "We still need to understand exactly
the mechanism for the effect," she said.
The tiny contact point between the wires forces the boundary of the magnetic
field to be very narrow, effectively blocking electrons, said Ross. "Hence
this phenomenon is a direct result of the small size of the contact,"
she said.
The ballistic magnetoresistance the researchers produced could be used
in practical applications in 4 to 6 years, said Chopra.
Chopra's research colleague was Susan Hua. They published the research
in the July 1, 2002 issue of the journal Physical Review B. The research
was funded by the National Science Foundation (NSF) and the Department
of Energy (DOE).
Timeline: 4-6 years
Funding: Government
TRN Categories: Data Storage Technology; Materials Science
and Engineering; Physics
Story Type: News
Related Elements: Technical paper, "Ballistic magnetoresistance
over 3000% in Ni nanocontacts at room temperature," Physical Review B,
July 1, 2002
Advertisements:
|
July
24/31, 2002
Page
One
Disks set to go ballistic
Two-step
queries bridge search and speech
Implant links
nerve cells to electronics
Silicon chips set to
go atomic
Light switch
promises powerful computers
News:
Research News Roundup
Research Watch blog
Features:
View from the High Ground Q&A
How It Works
RSS Feeds:
News | Blog
| Books 
Ad links:
Buy an ad link
Advertisements:
|
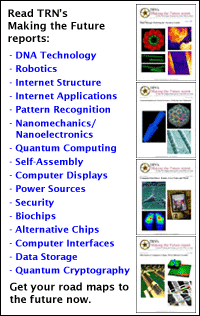
|
|
|