Implant
links nerve cells to electronics
By
Kimberly Patch,
Technology Research News
Although nerve cells and electronics work
quite differently, scientists have for decades been trying to connect
them.
Researchers from the University of Michigan have improved the connection
by coating electrodes with a carefully controlled mix of plastic and protein.
The work is a step toward achieving finer control of prosthetic limbs,
restoring damaged senses like sight and hearing, and providing direct
connections between the brain and equipment like cameras.
Electronics and neurons both communicate using electrical pulses, but
the physics involved in each is different. Electronics use currents of
electrons, which carry a negative charge, while neurons use ions, which
have an imbalance of electrons, and so can carry a negative or positive
charge.
Electronics generate pulses by using energy to move electrons along metal
wires. A nerve cell generates a pulse from one end of its oblong shape
to the other by using energy to pump positively-charged sodium, potassium,
or calcium ions across the membrane at one end, then allowing the ions
to flow back all at once. Nerve cells range in length from 10 microns
to 1 meter. A micron is one millionth of a meter.
The researchers' electrode coating is a mix of a polymer that conducts
electrical current and proteins that allow similar interactions with ions.
The polymer also contains a substance that encourages neurons to grow
around the metal electrode.
The key to the method is that the coating is not smooth, but has a fuzzy
surface that increases the contact area between electrode and brain tissue,
said David C. Martin, an associate professor of materials science and
engineering, and biomedical engineering at the University of Michigan.
"A large surface area can be packed into a small volume if the structure
is fuzzy," he said.
The fuzzy structure "makes it possible to accommodate the dramatic difference
in mechanical properties between the soft brain tissue and the hard silicon
devices," Martin said. More surface area provides more places where neurons
can bind to the electrode, gives neurons better access to the growth substance
contained in the polymer, and makes it easier for electric charge to move
across the interface, he said.
To test their method, the researchers coated electrodes a few millimeters
long, 100 microns wide and 15 microns deep, and encouraged rat glial cells
to grow on the devices. Glial cells provide support for neurons, which
conduct the impulses of biological communications systems. A micron is
one thousandth of a millimeter, and a human hair is about 75 microns in
diameter. They also implanted the probes into living guinea pigs.
The fuzzy surface allowed electric current to flow more easily at the
1-kilohertz frequency that corresponds to the 1-millisecond width, or
wavelength, of a neural pulse. The surface decreased the impedance, or
resistance to electron flow by one or two orders of magnitude, according
to Martin. "The lower the impedance at 1 kilohertz, the easier it is for
electrical information to transfer from the probe to cells or vice versa,"
he said.
As the coating gets thicker, its surface becomes more and more rough,
increasing the surface area, but there's also a point at which it becomes
too thick and begins to increase resistance, said Martin. "There is an
optimal film thickness at which the [charge] transport is the easiest,"
he said.
The experiments also showed that the coatings encouraged neurons to bind
to the electrodes. "We see neural cells attached to the surface of the
probe after it is removed, whereas uncoated probes come out clean," said
Martin.
The researchers are currently working on coating electrodes with softer
films of hydrogel materials that swell when they come in contact with
water, and could increase the surface area further, according to Martin.
They are also preparing to do tests with implanted probes in in rats.
The coatings could eventually be used for other types of devices, like
pacemakers, that interface electronically with living tissue, Martin said.
Eventually the technique could be used to make new connections between
electronics and tissue to restore sight or hearing, said Martin. "Another
possible outcome may be the ability to control robotic equipment, or prosthetic...
limbs."
This type of interface could also allow for direct brain interfaces to
cameras that detect things humans cannot, like infrared light or magnetic
fields, Martin said.
Martin's research colleagues were Yinghong Xiao, Junyan Yang, David Lin,
Donghwan Kim, and Xinyan Cui. They presented the research at the 34th
central regional meeting of the American Chemical Society on June 27 at
Eastern Michigan University. The research was funded by the the National
Institutes of Health (NIH) and the National Science Foundation (NSF).
Timeline: Unknown
Funding: Government
TRN Categories: Biotechnology; Materials Science and Engineering;
Human-Computer Interaction
Story Type: News
Related Elements: Technical paper, "Surface Modification
of Neural Recording Electrodes with Conducting Polymer/biomolecule Blends,"
Journal of Biomedical Materials Research, August, 2001 .
Advertisements:
|
July
24/31, 2002
Page
One
Disks set to go ballistic
Two-step
queries bridge search and speech
Implant links
nerve cells to electronics
Silicon chips set to
go atomic
Light switch
promises powerful computers
News:
Research News Roundup
Research Watch blog
Features:
View from the High Ground Q&A
How It Works
RSS Feeds:
News | Blog
| Books 
Ad links:
Buy an ad link
Advertisements:
|
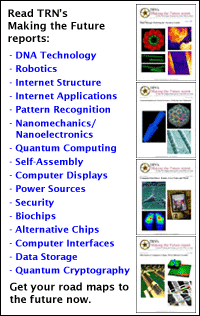
|
|
|